Magnetic Properties of Ceramic Minerals
By Paul Fears | 15 February 2021
Magnetic minerals and fine iron have plagued ceramic producers since the first glazed pot was produced many thousands of years ago. Early ceramicists adopted elaborate and colourful patterns to cover any imperfections caused by such contamination. Over time, the demand for white or single coloured ceramic products increased, making disguising any imperfection increasingly difficult.
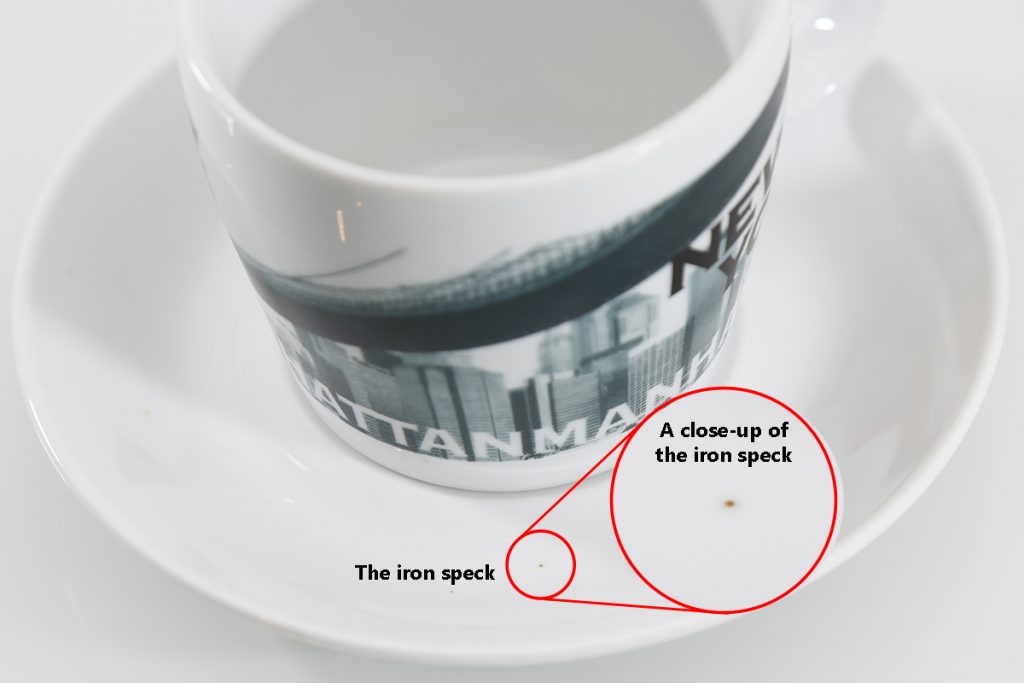
Ceramic producers turned to technology, with magnetic separators and screens, to identify and then remove the problematic minerals and fine iron. Many ceramic raw material deposits, such as feldspar, silica sand, ball clay and kaolin, have problematic magnetic minerals included micas (muscovite and biotite), hematite, chromite, and iron-stained quartz. Additionally, free iron is often introduced into the process from the wear or failure of process plant. Understanding the minerals’ magnetic properties and their reaction within a magnetic field defined the magnetic separator design process.
The behaviour minerals exhibit in a magnetic field is classed under one of five headings:
- Diamagnetic;
- Paramagnetic;
- Ferromagnetic;
- Anti-ferromagnetic;
- Ferrimagnetic;
These classifications define whether separation is possible with a magnetic separator.
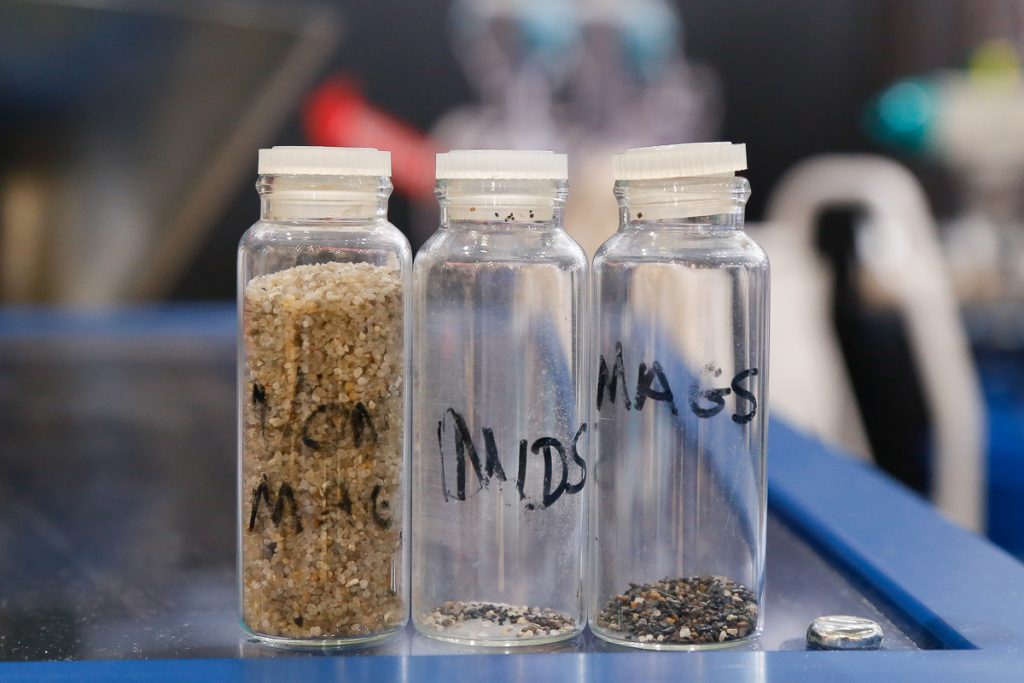
Source of Magnetic Properties
The magnetic properties of any mineral reside in the electrons of the atoms or ions. In accordance with the principles of wave-mechanics, the electron, moving in a closed path about the nucleus, is considered as a current behaving like a wave, and this moving current generates a magnetic field. When a crystal is placed in an external non-uniform magnetic field, there is a force working to align the magnetic fields of the atoms to produce a magnetic moment for the whole crystal. The magnetic susceptibility χ, is the ratio of the resulting magnetic moment, M, to the strength of the external field, H.
χ = M/H
Diamagnetism and Paramagnetism
Diamagnetic minerals have a small negative value of χ and are slightly repelled by the magnetic field. In contrast, paramagnetic minerals have a small positive value of χ and are weakly attracted by the field.
Paramagnetism is associated with the spins of the electrons, whereas diamagnetism is related to their distribution in space. Diamagnetism is a property possessed by all atoms. However, when the atom contains an odd number of electrons, or has incomplete electron shells (as in the transition elements) imbalance of the electron spins causes the paramagnetic effect to overshadow the diamagnetic part of the total magnetic susceptibility. Paramagnetism is also found in metals where there is a cloud of free conduction electrons.
This behaviour is only applied generally to crystals because the internal crystal field, as a whole modifies, the magnetic effects. The electronic energy levels in a crystal are described as being split and the total magnetic susceptibility depends on the distribution of the electrons in the different levels. Therefore, in complex compounds, it is not possible to predict the magnetic properties.
In minerals, iron-bearing structures are paramagnetic. However, there are paramagnetic minerals without iron. The differences in magnetic susceptibility are sufficient to enable separation using high-intensity magnetic separators.
Bismuth is the only one example of a diamagnetic mineral. Paramagnetic minerals are more widespread in ceramic feedstocks and include Hematite and Biotite Mica.
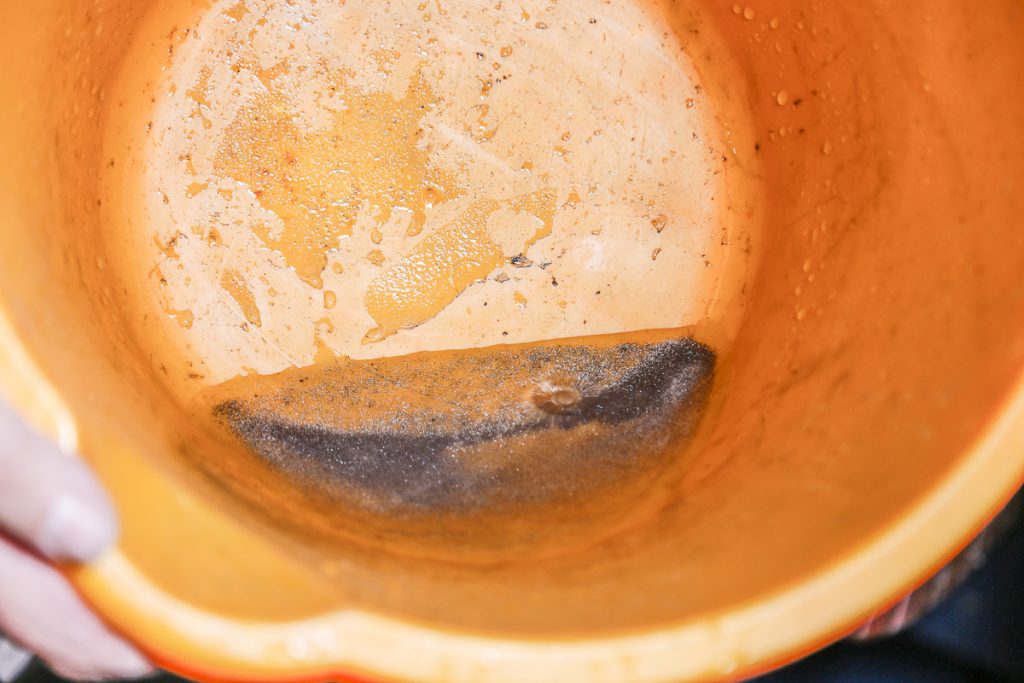
Ferromagnetism
Ferromagnetic minerals possess a magnetic moment even in the absence of an applied magnetic field. They are strongly attracted by even a weak magnetic field and remain permanently magnetised. However, ferromagnetic substances also exist in unmagnetised conditions when, at room temperature, the electronic magnetic moments are permanently in alignment as a result of interaction between neighbouring atoms.
Iron, Cobalt, Nickel and Pyrrhotite are typical examples of the ferromagnetic species.
Antiferromagnetism and Ferrimagnetism
The way in which electrons align in certain crystals produces either an antiferromagnetic or ferrimagnetic effect.
Antiferromagnetism
This occurs when adjacent atoms interact in a manner to align the spins in parallel but opposed directions called antiparallel spins. The two sets of moments cancel one another and there is no permanent magnetic moment. Typical examples include metals such as Chromium and oxides such as Nickel Oxide (NiO).
Ferrimagnetism
Antiparallel alignment, in which the components in opposite directions are not equal, results in a permanent moment which is ferrimagnetism. Magnetite is an important example of a ferrimagnetic mineral.
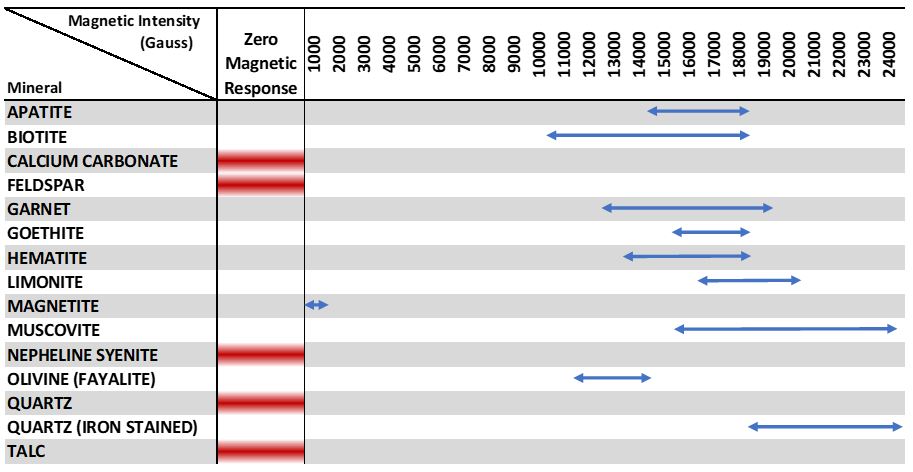
Consequence of Magnetic Particle Contamination
The presence of magnetic particles in ceramic body or glaze, both in mineral form or as free-iron, causes a wide range of issues during the firing processing. In the past, such flaws were covered by ornate and colourful glazes and decoration. However, modern ceramics producers do not have the luxury of implementing such methods.
Magnetic particles cause both structural and surface defects, as well as colour and brightness issues. Lower value, mass-produced ceramics often have very visible black spots and associated glaze deformation. Less visible black or brown particle specking is also common. Such defects occur when magnetic minerals or free-iron is present in the glaze during the firing process. The heat is absorbed and retained by the particle, causing a darkening in colour and expansion. The particle may also ‘pop’, causing defects in the surrounding glaze.
Another effect is the changing of the final fired glaze colour and darkening of the brightness, especially when white or off-white, due to the presence of fine magnetic particles.
Defects on ceramic tiles, tableware and sanitaryware, which rely on perfect appearance, result in either partial rework or total rejection and recycling. In plants with insufficient systems to remove the magnetic particles, such rates can be above 10%. This is costly in both time and materials.
Non-visible magnetic contamination remains problematic. Magnetic particles remaining in the body prior to firing experience the same reaction to heat as those in the glaze. This weakens the surrounding ceramic structure, resulting in fine cracks that develop over time. Failure of a ceramic product due to fracturing is commonly traced back to one or more magnetic particles, which are then visible along the fracture line. A single magnetic particle is enough to trigger such catastrophic failure.
This poses a risk for many high-performance ceramics, especially as the contamination is not visible from the outset. Failure only occurs over time.
Magnetic Separation of Minerals
Understanding the magnetic properties of the minerals in a particular ceramic raw material is vital when optimising magnetic separation efficiency. The wide range of magnetic separator designs use different strengths and types of magnetic field to produce a separation. Several designs of magnetic separator may feature in a single mineral processing operation.
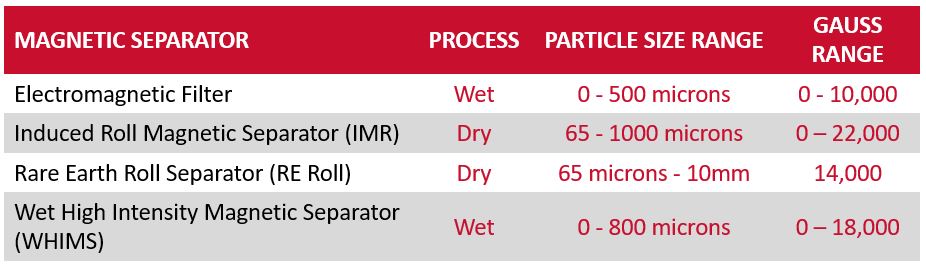
The range of high-intensity magnetic separators is divided into dry and wet processing. Many ceramic raw materials, such as feldspar, zircon and silica sand are handled and processed in a dry state. Most ceramic mineral processors magnetically treat their own reserves as higher specification materials generate increased revenue. High-intensity magnetic separators remove magnetic minerals and free iron. Designs include the Rare Earth Roll Magnetic Separator and Rare Earth Drum Magnet, which both utilise permanent magnets, or the electromagnetic Induced Roll Magnetic Separator, which is better suited for handling high-temperature materials (e.g. directly after drying).
Within the manufacturing plant, the spray dry ceramic process commonly introduces free fine iron into the process. Both the Rare Earth Roll Magnetic Separators and Drum Magnets are employed to remove such problematic contamination.
- Technical Product Information: Magnetic Separators used in the Ceramics Industry
Once the ceramic raw materials have been milled and mixed into slips, body and glazes, further magnetic extraction takes place. The milling process often liberates entrapped mineral iron, whilst free iron is reintroduced from the process (e.g. during screening, pumps, etc).
Electromagnetic Filters, producing high-magnetic fields, cleanse both the body and the glaze. Larger-sized models, for high capacities, provide the final separation stage for removing very fine and weakly magnetic minerals from body. Small-sized Electromagnetic Filters, often on mounted on moveable frames, separate fine iron and weak magnetics from the glaze. However, care is needed, as some glaze colouring is magnetic.
As a final check and to prevent any visible contamination on the glaze, neodymium rare earth Tube Magnets or Magnetic Liquid Traps with neodymium magnets are positioned as close to or within the glazing station. This captures any magnetic particles introduced to the glaze during transportation to the station.
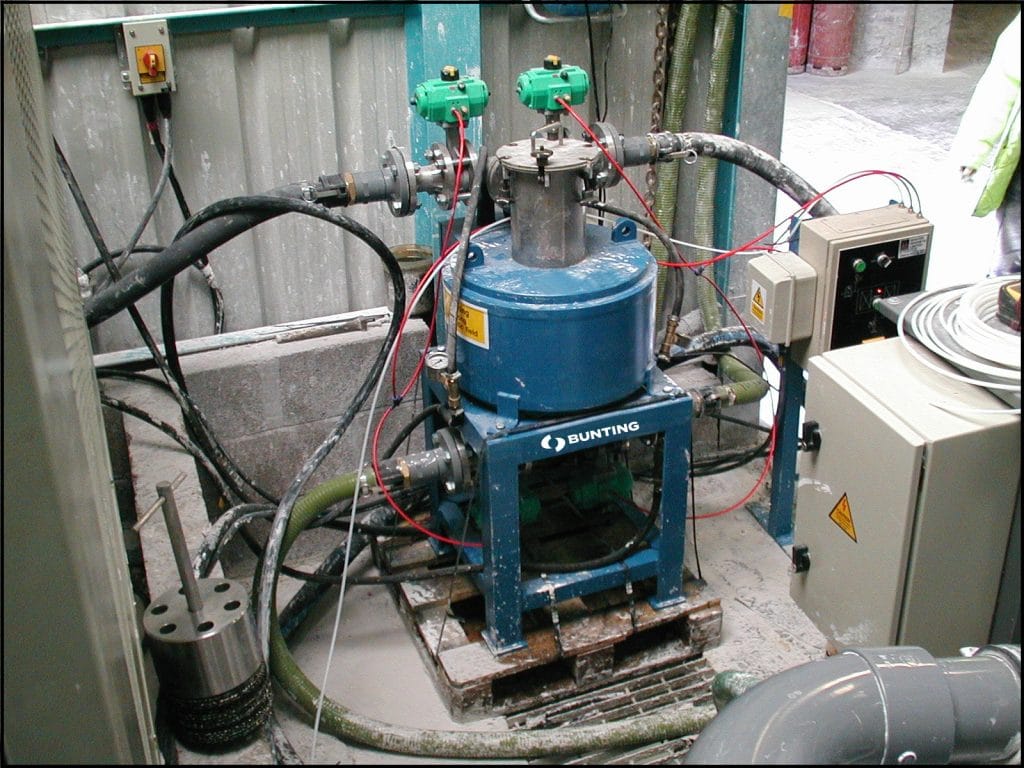
Future Mineral Reserves
As traditional ceramic mineral reserves are depleted and more complex deposits are mined, understanding the mineralogy in terms of magnetic susceptibility is vitally important. Magnetic separator suppliers work closely with mineral processors and plant designers, often at the project feasibility stage, providing advice on the optimum design of magnetic separator and stage within the process. This often involves extensive test work in magnetic separation laboratories and at site.
The ongoing demand for white and pattern-free ceramics means that there is no rest for ceramic producers and raw material suppliers in the battle to eradicate magnetic minerals and free iron.
Related Technical Articles
- Keeping Ceramic Tableware Iron Blemish Free
- Keeping Ceramic Sanitaryware Iron Free
- Keeping Ceramic Tiles Iron Free
Optimum Magnetic Separation Reduces Rejects
Understanding the ceramic manufacturing process is important when making recommendations for the optimum magnetic separation equipment. The magnetic separators mentioned in this review are used successfully in ceramic production operations. However, each manufacturing process is unique and a visit by a local Bunting Sales Engineer will help understand a specific process and iron contamination issue and propose a specific solution.
For additional information on eradicating iron contamination blemishes in ceramic tableware, tiles, or sanitaryware, please contact us on:
Email: Gordon Kerr at GKerr@buntingmagnetics.com
Telephone: +44 (0) 1527 65858
Follow us on social media